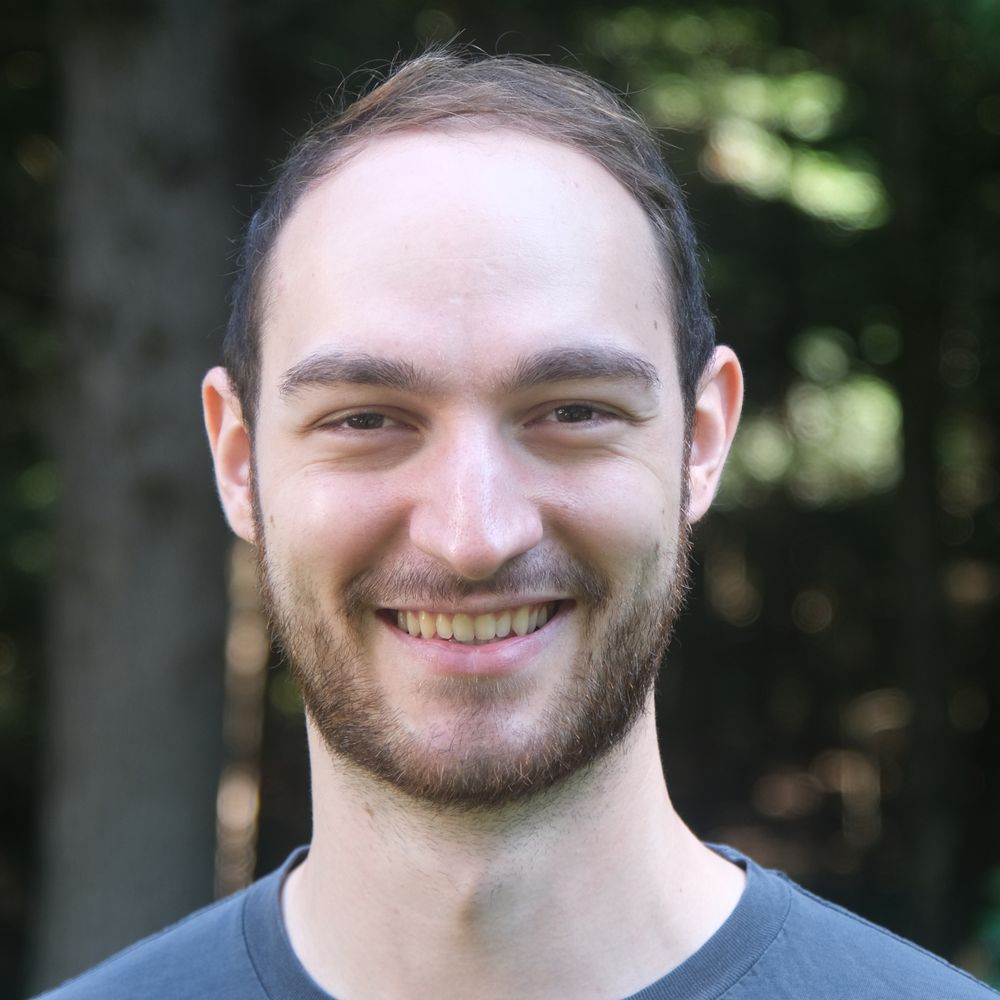
Joe DeMartini
University of Maryland
Discrete Element Models of Low-Gravity Planetary Surfaces
Since the turn of the millenium, planetary scientists have widely accepted that most asteroids in the size range of 100 m to 10 km are gravitational aggregates of pebble- to boulder-size fragments called “rubble piles,” rather than monoliths. Rubble-pile asteroids are suspected to be particularly common among the “Near-Earth” and “Potentially Hazardous” asteroid populations, which are the most accessible and exigent for space missions like the recent Hayabusa2, OSIRIS-REx, and DART missions. NASA’s Planetary Defense Coordination Office maintains that impacts of small solar system bodies are some of the only preventable natural disasters for Earth, and these rubble piles likely represent a large portion of those impactors which may need to be deflected in the future. Currently, the largest sources of uncertainty for deflection missions are the surface and near-surface structure and energy dissipation in the target body, which are dependent on material properties such as the strength, grain size distribution, and porosity of the regolith (dust, rocks, sand, boulders, and pebbles) systems that makes up these rubble piles.
My research focuses primarily on the development and implementation of the parallelized, N-body gravity tree, and soft-sphere discrete element method code PKDGRAV in the context of modeling the dynamics of granular surfaces on small solar system bodies. I model rubble piles and smaller regolith beds as systems of individual spheres with material properties (mass, density, friction) akin to the fragments that make up asteroids and the surfaces of those and other small solar system bodies like the Moon. Recent developments that I have worked on include the efficient modeling of irregularly shaped particles made by “gluing” spheres together (see Fig. 1a). Our preliminary results show, on a large scale, that modeling with irregular particle shapes (Fig. 1b) can increase the porosity of a rubble pile while also increasing its bulk strength: with fewer particles packed more “precariously” than in the spherical case, a rubble pile can spin faster before breaking apart, and is more resistant to tidal- and impact-induced rupture. On the scale of a regolith surface, we can also create stable, high-porosity (low density; a lot of void space between particles) granular beds in low-gravity using irregular particle shapes, which more accurately mimic the conditions that the OSIRIS-REx sample return mechanism experienced when it touched the surface of Bennu. With these high porosity beds, we can model interesting phenomena like the Brazil-nut Effect, where large grains rise to the surface of granular systems after vigorous shaking, despite having equal or higher density compared to the other grains in the medium, and which I am finding can be much more efficient when modeled with irregularly shaped particles, depending on their shapes (Fig. 1c).
Figures 1. a) A few examples of irregular particle shapes made by “gluing” PKDGRAV spheres together. b) A roughly-spherical rubble pile constructed from mixed irregularly shaped particles. c) A system of irregularly shaped particles after vigorous shaking, with the large (red) grain having risen to the top of the medium